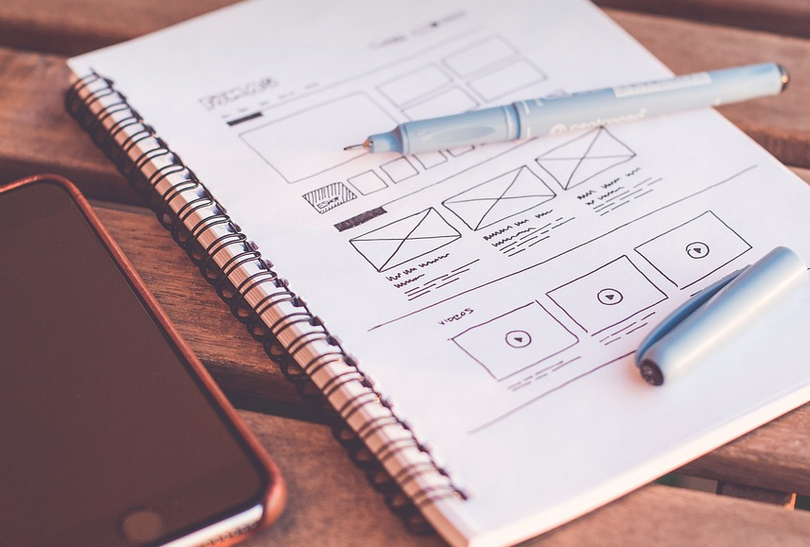
A Journey into the Core of Energy and Heat
Dive into the fascinating world of thermodynamics, where energy and its transformation play a central role in our daily lives. This deep dive explores the fundamental principles of thermodynamics, providing engineers with a comprehensive understanding of how systems interact with heat and work. Prepare to unlock the secrets behind the efficiency of engines, the control of chemical processes, and the design of sustainable power plants!
Thermodynamics, often referred to as the “science of energy,” forms the bedrock of many fields, including engineering, physics, chemistry, and even biology. This branch of science delves into the relationship between heat, work, temperature, and other key thermodynamic quantities.
Imagine a steam engine powering a train, or a car’s internal combustion engine – these are examples of energy transformation in action. Thermodynamics provides us with tools to analyze and optimize such processes, ensuring maximum efficiency and minimal waste. Understanding the principles behind how heat flows from one system to another is crucial for designing efficient devices and systems.
At its core, thermodynamics revolves around a set of fundamental laws that govern energy exchange in physical systems:
- First Law: The conservation of energy. Energy can neither be created nor destroyed, only transferred or transformed from one form to another.
- Second Law: Entropy increases over time. This law explains why certain processes are irreversible and that nature tends towards disorder.
- Third Law: Absolute zero is unattainable. As a system approaches absolute zero temperature, its entropy approaches a minimum value, but it never actually reaches this point.
Let’s explore the first law in more detail, as it clarifies how energy transfer works in any practical scenario:
The First Law of Thermodynamics states that the change in internal energy (ΔU) of a closed system equals the heat added to the system (Q) minus the work done by the system (W). Mathematically, this is expressed as:
ΔU = Q – W
This equation tells us that the change in internal energy of a system equals the energy transferred to or from it by heat (Q), minus the energy used to do work (W). In simpler terms, if we add heat (Q) to a system, its internal energy increases. However, if the system does work (W) on the environment, some of this added energy will be converted into other forms like mechanical energy.
To illustrate, consider a gas expanding against atmospheric pressure in an engine. This expansion work done by the piston is related to heat transfer – as the gas expands, it absorbs some heat from the combustion process, causing an increase in the internal energy of the gas molecules.
As we delve deeper into thermodynamics, understanding these fundamental principles will be crucial for a deeper comprehension of how engines transform chemical energy into mechanical work. We’ll learn about various thermodynamic cycles like Carnot cycle and Rankine cycle, which are central to engineering applications.
Exploring the Principles: A Closer Look at Key Concepts
Within the realm of thermodynamics, numerous concepts hold key importance for engineers. Let’s delve into a few:
**1. Temperature:** One of the most fundamental thermodynamic concepts is temperature, which represents the average kinetic energy of particles within a system.
Temperature scales measure this energy in different units: Celsius (°C), Fahrenheit (°F), or Kelvin (K). For engineers’ applications, understanding temperature is vital for designing and operating systems that work efficiently.
**2. Heat:** Heat is the transfer of thermal energy between objects due to a difference in temperature. Heat always flows from hotter objects to colder ones, driven by the tendency to equilibrium.
In engineering applications, understanding heat transfer mechanisms like conduction, convection, and radiation is crucial for designing efficient systems – for example, heat-exchange components in machinery are designed using principles of these three heat transfer modes.
**3. Work:** This refers to the energy transferred when a force causes a displacement. In engineering contexts, work is crucial for understanding how mechanical systems function. For instance, understanding work done by an engine piston relates directly to its ability to generate power.
Engineering applications of work often involve engines and machines that use forces to convert energy into useful work – this can range from simple hand pumps to complex industrial machinery like robots. Understanding the principles of work allows engineers to design more efficient systems for driving these machines.
**4. Entropy:** This refers to a measure of disorder or randomness in a system. Entropy increases when energy is dispersed, leading to degradation and loss of useful function. For engineers:
Understanding entropy impacts the efficiency of various processes such as engine combustion or chemical reactions. The Second Law of Thermodynamics states that entropy always increases in an isolated system.
**5. Efficiency:** This quantifies how effectively a device converts energy from one form to another. For engineers, understanding the concept of overall and specific process efficiencies helps optimize systems for maximum output and minimal waste.
Examples include the use of efficient power generation equipment, reducing energy loss in transport systems (like vehicles or trains), optimizing processes that convert materials into usable products. Efficiency plays a crucial role in ensuring sustainability and minimizing environmental impact.
*It’s important to note that these concepts provide just a glimpse into the vast world of thermodynamics.*
A Practical Application: The Efficiency of Engines
Let’s consider the internal combustion engine as an example – one of the most prevalent types of engines used in vehicles. The efficiency of an engine, which determines how much of the chemical energy in fuel is converted into useful work (like movement), plays a vital role in energy conservation and environmental sustainability.
To maximize engine efficiency, engineers employ several strategies:
* **Fuel-Air Mix Optimization:** Precise control over the air-to-fuel ratio ensures optimal combustion for maximum power output while minimizing waste. * **Engine Tuning:** Adjustments to ignition timing and compression ratios can improve both power and fuel efficiency. * **Exhaust System Design:** Optimizing exhaust flow patterns enhances combustion efficiency, reducing wasted energy. * **Cooling Systems:** Efficient cooling systems prevent overheating, ensuring optimal engine performance while minimizing wear and tear.
These strategies highlight the significance of thermodynamics in improving engine design and enhancing overall efficiency. Understanding how these principles influence real-world applications allows engineers to implement innovative solutions for developing more efficient and sustainable vehicles.
The Next Steps: Delving Deeper into Thermodynamics
This journey through the fundamentals of thermodynamics provides a solid foundation for further exploration. For those looking to deepen their understanding, several resources can provide valuable insights:
* **Advanced Books and Textbooks:** Explore specialized texts that delve deeper into specific aspects like fluid mechanics, statistical thermodynamics, or chemical reaction engineering. * **Online Courses and Resources**: Online platforms offer courses and tutorials focusing on different areas of thermodynamics – some are even available for free! These resources can enhance your comprehension and provide practical applications of the concepts learned in this article.
No matter your level, embracing the principles of thermodynamics opens doors to a wider understanding of the world around us. Whether you’re a student pursuing an engineering degree or an experienced professional seeking deeper insights into energy systems, exploring this fascinating field will undoubtedly provide invaluable knowledge and skills.